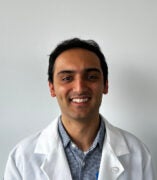
Arvind Draffen
2024 students
Email:
2024 CIP Week 1: Neurosurgery
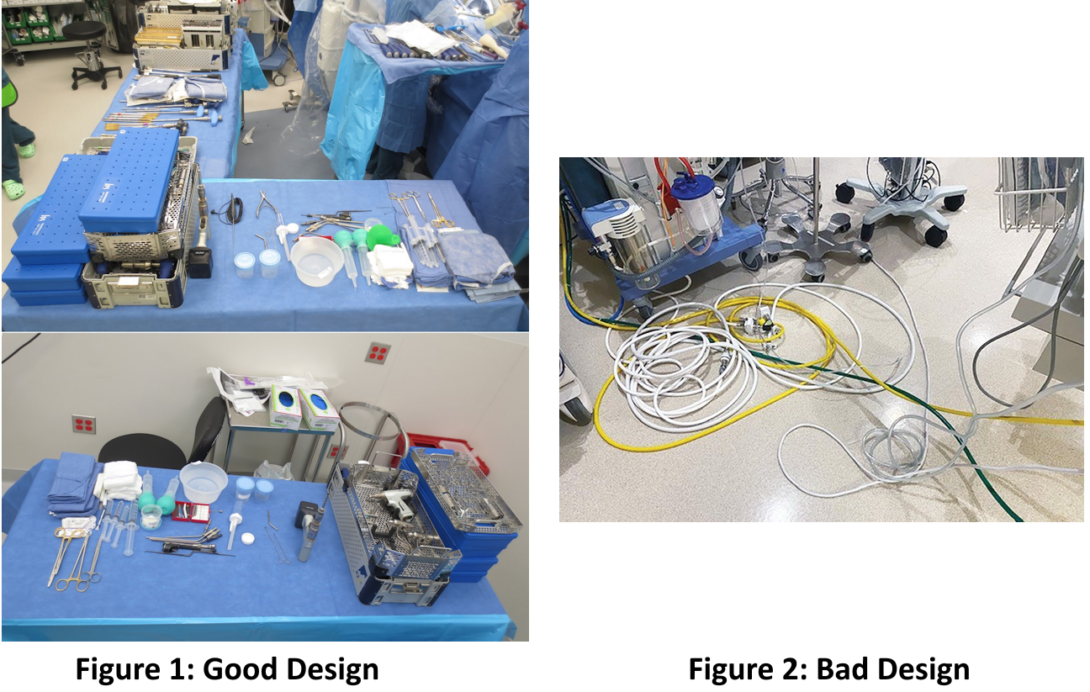
This first week of the Innovation Medicine (IMED) Clinical Immersion Program (CIP), our group of 3 rising M2 medical students and 2 rising senior biomedical engineering students gained some of our first experiences in the operating room, specifically the neurosurgery OR, with Dr. Mehta. In this time, we were adjusting to the new environment of the OR, acclimating to the pace and space of this new setting. On Monday, Wednesday, and Thursday I primarily spent my time in the OR, observing primarily spinal procedures including TLIFs, as well as a posterior pituitary tumor excision. Tuesday gave me the opportunity to listen in on morning neurosurgery rounds before observing a cranial angiogram. Both a benefit and drawback of not having covered much neuroanatomy curriculum yet meant that I was able to, for the most part, feel very unfamiliar with the medical and nonmedical context surrounding me during this first week. While I could follow very little of the complex clinical reasoning, this presented the advantage of preventing me from considering things how they “should” be and, instead, observing things how they were, without any additional learned biases and expectations. Throughout the week, I worked together with the team to absorb impressions of technologies, workflows, and designs in general – looking for those that seemed thoughtful and well-engineered and for those that seemed more cumbersome. A highlight of those thoughts from the first week are presented in the following sections.
Good Design:
A good design I observed was of the neurosurgical equipment sets used in surgeries I observed. These surgeries involved removal or installation of screws, cages, or other devices, particularly into the spinal cord, and each device has specific associated installation equipment. Tasks such as placement of the device into a very specific location, tightening of screws, or tactile feedback of placement required high precision, and a wide array of supporting equipment to perform correctly. The neurosurgical equipment sets featured many modular designs, with interchangeable handles and attachments. The equipment came preassembled in multiple specific kits that were arranged by the scrub tech, and provided to the surgeons at their request, helping to reduce time spent on locating tools during the procedure, allowing the surgeons and scrub tech to exchange tools more efficiently. Modularity of the toolkits also allowed for additional procedure and company specific devices to work alongside the “standard” toolkit for a specific hospital, as certain devices require specialized tooling for their installation and removal.
Organization of the equipment also allowed for faster inventory, both preoperatively and postoperatively, where the latter is particularly imperative to ensure all equipment is accounted for prior to closure of the surgical site. Of note, although the modularity of tooling allows for very high per-procedure specificity, it is also accompanied by a significant learning curve. Due to the specific nature of company-specific devices, company technicians were present in each of the spinal surgeries I observed, assisting the scrub tech with assembly and disassembly of the tools and advising the surgeon on installation specifics. For scrub techs less experienced in certain procedures, some tools being slightly misplaced required additional time to locate due to the plethora of tools laid out across the table.
Bad Design:
In the OR, there can be movement into and out of the operating room, especially knowing that surgery requires a sterile field within which the operation takes place. While the sterile field was preserved, the periphery of the room was not sterile, and allowed for movement between connected rooms, as well as into and outside of the room itself. This, of course, allows for additional interventions such as portable imaging to be performed to confirm placement during the surgery, as this can be performed from outside the sterile field. Since the non-sterile portion of the room is mostly a peripheral ring around the central sterile field, this is a narrow space, allowing for two people to walk by each other if they turn sideways. This area can become congested quite easily, not only if there are additional people in the room , but due to the equipment (such as computers, monitoring devices, imaging) that assist with the operation. This area experiences substantial foot traffic throughout the operation, as staff move throughout the OR to assist in the procedure by performing tasks such as providing additional equipment to the sterile field (e.g. cloths, devices), people entering or leaving the procedure after scrubbing in and out, or documenting elements of the procedure as it is ongoing.
A particular element of this small space I noted were a substantial amount of “trip hazards” strewn along the floor, particularly cables for many of the powered devices using in the procedure (such as surgical suction devices, vitals monitoring). Staff walking through the space were always having to take extra care and attention walking along the perimeter of the OR as to not trip or dislodge any cables along the floor, with the possible risk of unplugging a critical cable or toppling a table with medical equipment, especially when moving large devices such as a C-arm or O-arm into and out of the OR.
The newer Specialty Care Building (SCB) has alleviated many of the concerns of cables across the floor by having much of the cable management being ran from the ceiling rather than outlets low on the walls. This allowed for much of the cable management to be away from high-traffic areas within the OR and reduce the possible associated hazards.
2024 CIP Week 2: Neurosurgery
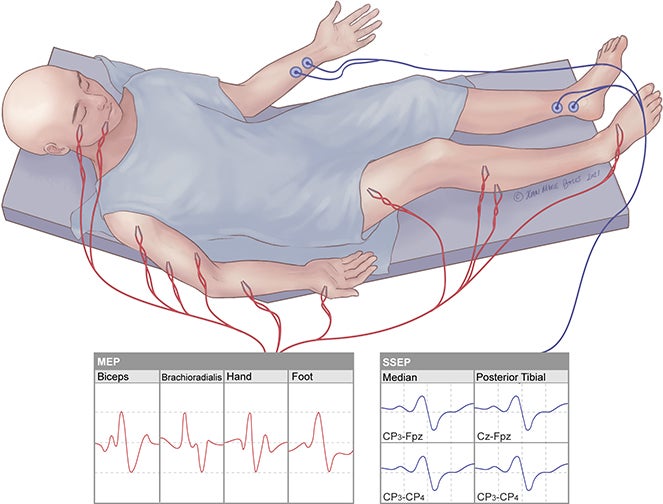
One of the neurosurgery procedures I observed this week was of a craniotomy for an intracranial neoplasm, and subsequent removal of the tumor. As part of the background for this procedure, I was able to learn about brain mapping and importance characterizing the location and potentially affected brain regions secondary to a neoplasm of the brain. One upcoming technology mentioned during my observation was high-fidelity brain mapping software, in this case provided by the company Omniscient Neurotechnology [1]. This software was more adjacent to the procedure itself, but offered extremely detailed maps of the brain, allowing stratification and selective visualization by features such as, for example, brain network types (e.g. sensory, motor), offering the potential to assist in preoperative planning.
A key element of the craniotomy using practically during the procedure for characterizing the tumor was cortical stimulation, which uses electrical stimulation to localize the function of specific regions of the brain. This procedure is invasive and can be performed intraoperatively once the segment of the skull has been removed and the dura mater peeled back, after which a probe is placed on the brain and an electrical impulse is transmitted. The impulse creates a reversible lesion that can produce a testable response, allowing characterization of the electrically simulated area. In this procedure, the patient was anesthetized using general anesthesia, and motor mapping was performed through use of electrodes placed in key sites of measurement on the head and extremities. The commercial solution used in the OR for this procedure was the Cadwell Cascade IOMAX [2]. This solution provides a base module, 32-channel amplifier, an LCSwap switch matrix to record direct cortical stimulation, and limb modules for recording and stimulating the arms and legs. The LCSwap was used in this procedure for direct cortical stimulation, and motor responses (e.g. brachioradialis) were monitored by the limb module.
A specific difficulty I noted during this part of the procedure was in inducing a measurable response. At a specific site, impulses were sent multiple times, with increasing current levels, to attempt to “threshold” the response, but were ultimately unsuccessful in some regions. The type of probe was subsequently changed from a more precise type to a ball probe with a larger surface area. This required constant back-and-forth communication between the neurosurgeon and the neurophysiological monitoring specialist, including multiple rounds of removing and placing the probe in the same place to threshold many repeated “handshaking” procedures of confirming whether or not the probe was placed or removed. The cortical stimulation procedure requires a balance of sending a powerful enough impulse to induce a response, but not one too powerful to inflict damage. In doing additional reading, I came across one paper that, while focusing on awake cortical stimulation, sought to investigate connections between cortical stimulation and frequency of perioperative seizures [3]. This study was a retrospective chart review of 83 patients who underwent awake craniotomy with electrocorticography data, and concluded that intraoperative cortical stimulation is generally safe; however, they found that the electrical stimulation duration (more than 145 seconds) and number of stimulations (more than 60 total) were correlated with a significantly higher rate of afterdischarge seizures. Understanding that an increase in the number of electrical stimulations has potential to cause an increase in adverse outcomes in patients, as well as the corresponding communication overhead on neurosurgery staff and subsequent repeated stimulations also contributing to longer operation times, this provides an opportunity for further investigation of the current landscape for potential solutions.
References
[1] Omniscient Neurotechnology. Hyperpersonalized Brain Care. Published 2024. Accessed July 4, 2024. https://www.o8t.com/.
[2] Cadwell Industries Inc. Cadwell Cascade IOMAX Intraoperative Neuromonitoring Solution. Published 2024. Accessed July 4, 2024. https://www.cadwell.com/cascade-iomax/.
[3] Larkin CJ, Yerneni K, Karras CL, Abecassis ZA, Zhou G, Zelano C, Selner AN, Templer JW, Tate MC. Impact of intraoperative direct cortical stimulation dynamics on perioperative seizures and afterdischarge frequency in patients undergoing awake craniotomy. J Neurosurg. 2022 Apr 29;137(6):1853-1861. doi: 10.3171/2022.3.JNS226. PMID: 35535844.
[4] Brigham and Women’s Hospital. Intraoperative Neuromonitoring – Bi Lab. Published 2024. Accessed July 4, 2024. https://skullbase.bwh.harvard.edu/ionm/.
2024 CIP Week 3: Neurosurgery
The observations from my OR experience last week involving cortical stimulation mapping, as well as an additional case this week, prompted me to continue my research into issues related to this process. CNS tumors are mapped for a variety of critical regions, and can involve both surgical planning and understanding functional implications associated with tumor resection. In the two cases I observed, the patient was under general anesthesia for both, and cortical stimulation was primarily performed to understand the functional impact of the tumor (e.g. by assessing motor responses) and assessing the cortical excitability through direct probe stimulation. Excessive stimulation can lead to adverse effects, as well as brief in-operation seizures preventing immediate re-stimulation until the neurons have returned to their baseline state (in these latter cases, the neurons have become excessively excited and will not respond to any additional stimulation).
There are a variety of probe types, and although each serves a specific purpose, the goal of each would be to minimize current spread through the use of techniques such as shielding. Improved shielding would theoretically allow for lower voltage/current levels and more precise stimulation of specific areas, potentially reducing the number of stimulations if areas can more accurately be characterized through stimulation. Both of these factors are implicated in increased seizure risk [1]. The benefits of shielding can also possibly extend to non-surgical therapies, such as deep brain magnetic stimulation for neurological disorders including Alzheimer’s and Parkinson’s diseases, where otherwise the signal decay would render such a therapy ineffective [2]. Navigated transcranial magnetic stimulation can be used preoperatively as additional resources, but currently lacks the accuracy and specificity to be used to replace intraoperative cortical electrical stimulation [3].
One company that makes probes is Cadwell, of which one such design is their disposable bent ball tip probe [4]. This probe provides a bending feature for access under the microscope, and describes insulation to prevent current shunting, but does not offer further quantifiable specifics in the product description. Another company offering solutions in this area is Natus, who among other products, sell a bipolar probe for functional brain mapping [5]. This product page is also light on details, but does state the ball tips are gold-plated and that the probes have a 5mm distance between them.
Due to the broad scope of potential benefits to patients and physicians with shielding improvement, I decided to use this problem as the baseline for the development of a needs statement. Iterations of potential needs statements, as well as the rationale behind changes, will be described in the following sections.
Iteration 1:
OR staff require a cortical stimulation probe with better shielding to improve stimulation accuracy.
- Population: OR staff (surgeons, stimulation technicians)
- Opportunity: Cortical stimulation probe with better shielding
- Outcome: Improve stimulation accuracy
Rationale for changes:
- This iteration was somewhat vague on the population mentioned. There are many different individuals in the OR with specific roles, and thus it would be better to be more specific as to who specifically would be affected by this need.
- This iteration provides a solution as the problem (e.g. suggesting the solution for better shielding). It will be better to be more open-ended about the problem and allow the solution to be formed from more extensive research, design, and prototyping.
- The outcome is desirable, but also somewhat vague and not sufficiently measurable.
Iteration 2:
Neurosurgeons and stimulation technicians performing interoperative cortical stimulation need more accurate probes to reduce intra-operative and post-operative seizure occurrence rates.
References
[1] Larkin CJ, Yerneni K, Karras CL, Abecassis ZA, Zhou G, Zelano C, Selner AN, Templer JW, Tate MC. Impact of intraoperative direct cortical stimulation dynamics on perioperative seizures and afterdischarge frequency in patients undergoing awake craniotomy. J Neurosurg. 2022 Apr 29;137(6):1853-1861. doi: 10.3171/2022.3.JNS226. PMID: 35535844.
[2] Abu Yosef R, Sultan K, Mobashsher AT, Zare F, Mills PC, Abbosh A. Shielded Cone Coil Array for Non-Invasive Deep Brain Magnetic Stimulation. Biosensors (Basel). 2024 Jan 9;14(1):32. doi: 10.3390/bios14010032. PMID: 38248409; PMCID: PMC10813362.
[3] Al-Adli NN, Young JS, Sibih YE, Berger MS. Technical Aspects of Motor and Language Mapping in Glioma Patients. Cancers (Basel). 2023 Apr 6;15(7):2173. doi: 10.3390/cancers15072173. PMID: 37046834; PMCID: PMC10093517.
[5] https://www.natusmedicalstore.com/s/product/detail/01t5w00000FVsmlAAD
2024 CIP Week 4: Neurosurgery
Iteration of Prior Needs Statement
The final iteration from last week of my needs statement was as follows:
Neurosurgeons and stimulation technicians performing interoperative cortical stimulation need more accurate probes to reduce intra-operative and post-operative seizure occurrence rates.
To further assess this needs statements, I considered the IDEO model for innovation, which consists of three primary components: desirability, feasibility, and viability.
For the desirability element, I spoke with our mentor, Dr. Mehta, about his experiences with cortical stimulation probes. He shared with us that his experiences with the probes have been generally positive, and he has not had notable issues with their use in the operations he has performed using them. This, to some extent, decreases the desirability component of such a project, as the margin for improvement may be lesser than originally intended or hoped.
The feasibility and viability of such a project would depend on materials engineering and the gains that can be made in the design of the probe to reduce current spread. An avenue for improvement could consider exploring non-invasive cortical stimulation mapping, such as through the use of magnetic fields, which has been proposed in some papers, such as by Abu Yosef, et. al [1]. This could yield a benefit not only in reducing effects from current spread or increased required current, but also provide opportunities both for invasive and non-invasive mapping, leading to increased accessibility of the technology. While development of a magnetic-based solution may be desirable, it provides additional challenges that could decrease the viability of being able to create a solution that maintains the accuracy compared to other invasive alternatives that are currently used.
Additionally, in order to assess a design compared to other existing alternatives and iteratively within development, the criteria for improvement should be specific and testable. This would lead to a slight modification in wording of the outcome of the needs statement.
After considering the IDEO design criteria and lessons learned from the weekly CIP workshop, I have revised my needs statement to the following:
Neurosurgeons and stimulations technicians performing cortical stimulation mapping require probes with lower measurable current spread to reduce intra-operative and post-operative seizure occurrence rates.
Development of a New Needs Statement
In addition to considering previous needs statements during this week, our group continued to observe in the neurosurgery OR and find additional needs for possible innovation. A procedure I observed once again this week was a craniotomy for a tumor resection. This procedure used a MedTronic StealthStation for pre-operative navigation as is typically done for these procedures in the UIC neurosurgery OR. I also observed the use of a doppler ultrasound on the brain tissue, exposed after partial removal of the skull. In my secondary research and talking with Dr. Mehta at our weekly meetings, I learned this was to assess for a phenomenon known as “brain shift”, where soft tissue structures move during a procedure, both after removal of supportive structures such as bone and after resection of adjacent soft tissue structures such a tumor [2]. As a consequence, the initial pre-operative mapping is no longer completely accurate as it is not updated during the procedure as these shifts occur. One solution to combat this was the use of doppler ultrasound, as I observed during the procedure this week. This is the solution employed by MedTronic with their StealthStation, as the ultrasound data can be integrated into the pre-operative measurements. In performing secondary research; however, some papers report ultrasound data as being poor in accuracy, and propose alternative solutions such as intra-operative imaging with a single camera or multiple cameras for additional depth and contour data [2,3].
These observations and primary and secondary research led to the development of the following needs statement:
Neurosurgeons performing navigation-aided craniotomies need real-time feedback of soft tissue movement to reduce complications from unintended excision of tissue from pre-procedure mapping deviations and decrease procedure time.
References
[1] Abu Yosef R, Sultan K, Mobashsher AT, Zare F, Mills PC, Abbosh A. Shielded Cone Coil Array for Non-Invasive Deep Brain Magnetic Stimulation. Biosensors (Basel). 2024 Jan 9;14(1):32. doi: 10.3390/bios14010032. PMID: 38248409; PMCID: PMC10813362.
[2] Nabavi A, Black PM, Gering DT, Westin CF, Mehta V, Pergolizzi RS Jr, Ferrant M, Warfield SK, Hata N, Schwartz RB, Wells WM 3rd, Kikinis R, Jolesz FA. Serial intraoperative magnetic resonance imaging of brain shift. Neurosurgery. 2001 Apr;48(4):787-97; discussion 797-8. doi: 10.1097/00006123-200104000-00019. PMID: 11322439.
[3] Morin F, Courtecuisse H, Reinertsen I, Le Lann F, Palombi O, Payan Y, Chabanas M. Brain-shift compensation using intraoperative ultrasound and constraint-based biomechanical simulation. Med Image Anal. 2017 Aug;40:133-153. doi: 10.1016/j.media.2017.06.003. Epub 2017 Jun 15. PMID: 28651099.
2024 CIP Week 5: Neurosurgery
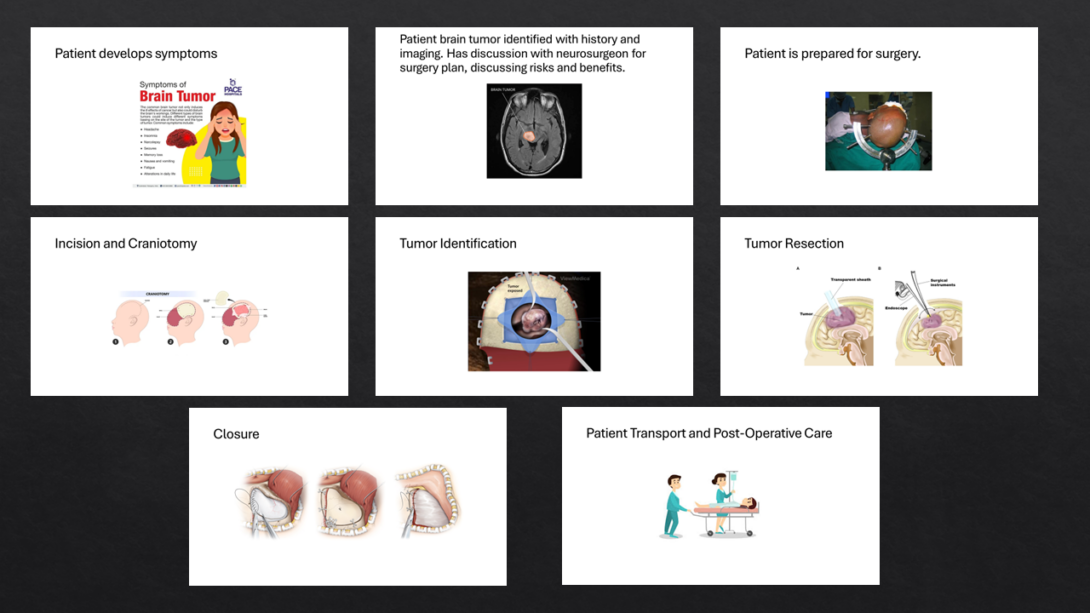
One of this week’s goals was to “zoom out” from a sometimes hyperfocused observation perspective and consider how observations made fit into the environment and circumstances by which they are surrounded. In addition to ensuring “fit” within an overall idea, this can also help to understand factors contributing to the observation. It also can provide an understanding of how seemingly similar situations can result in different outcomes due to decisions made at key inflection points. One such technique for this process is storyboarding, where we follow a case from a specific perspective, and attempt to gain insight into what decisions were made and how they resulted in the outcomes observed. I decided to follow-up on a needs statement I identified last week with a craniotomy brain tumor resection and possible soft tissue shifts. As part of this storyboard, I took a more macro approach to the overall procedure, considering the main steps of the procedure, and features of each step that I observed. The primary steps from my observation are outlined in the following storyboard image and accompanying commentary.
Step 1: Patient Develops Symptoms and is Evaluated.
The first step of a craniotomy for a tumor resection occurs well before the initial surgery. Someone who experiences a brain tumor may experience a variety of symptoms, depending on the type and location of the tumor, as well as the individual patient’s experience. Some possible symptoms include headache, insomnia, seizures, or fatigue [1].
Step 2: Patient Brain Tumor Identified with History and Imaging. Plan for Surgery Developed.
After experiencing these symptoms, the patient may seek the medical advice of a physician, who decides to pursue further evaluation. Imaging may be done, and this can reveal the tumor. The type of imaging depends on the tumor and associated clinical criteria depending on the history and physical exam. Common imaging performed may include a CT or MRI scan [9]. The physician and patient will subsequently have a discussion on developing a plan for management and treatment. This preoperative imaging may also be used for navigation for the operation, helping guide the surgical approach through intraoperative navigation. Should a craniotomy for resection of the tumor be clinically indicated, depending on its location, type, and the patient, this treatment option will be discussed. If the patient agrees to the procedure, depending on their understanding of the risks and benefits as explained by the physician, then the process continues to the next step of this linear workflow. This is a potential inflection point in this timeline, where a patient may not feel comfortable with such an invasive procedure and would prefer to pursue alternative treatment options.
Step 3: Patient is Prepped for Surgery
In the procedure I observed, the patient will have made the informed decision to undergo a craniotomy and scheduled their surgery. On the day of the surgery, the patient will likely have preoperative instructions they are to follow, such as no intake of food or water some hours prior to the operation. After this has been confirmed, the patient is subsequently prepared for surgery. The patient will then be administered anesthesia and other medications, and then properly positioned on the operating room table. This often requires flipping the patient from their initial bed to the OR bed, which takes multiple personnel and careful coordination. The patient’s head will then need to be firmly fixed in place, typically by a Mayfield clamp, which provides skull stabilization to prevent any movement throughout the surgery. A timeout is then performed, to prevent miscommunication and incorrect surgery.
Step 4: Incision and Craniotomy
The craniotomy is the process of removing the skull, allowing access to the site of the tumor for subsequent resection. This process involves drilling of multiple holes in the skull using a drill to the appropriate depth of the skull, followed by cuts to remove the portion of the skull covering the tumor and surrounding brain tissue. The craniotomy approach can be aided by surgical navigation, which fuses data from a scan immediately prior to the initial incision with preoperative imaging data, to help direct the optimal approach.
Step 5: Tumor Identification
The area surrounding the tumor is now accessible, and the borders of the tumor that can be resected must now be precisely identified. Preoperative imaging is used for approximation of the tumor borders, but depending on the type and location of the tumor, discrete border edges may not be visible, or resection may cause additional complications such as bleeding if near highly vascular tissue. One technique that can be used to characterize the tissue constituting the tumor is cortical stimulation mapping, which prompted my broader perspective of the entire procedure. The neoplasm may also often be visible through visual inspection.
Step 6: Tumor Resection
Now that the tumor is identified and accessible, the next step of removal of as much of the tumor as is safely possible. Ideally, the tumor will be very well demarcated and easily resectable, but this may not always be the case. The tumor could be tightly adhered to surrounding structures, or behind tissue that cannot safely be removed during the surgery. In these cases, as much of the tumor that can be removed without causing further complications is done, and then additional treatment options will be used for further management. This can also change the approach used for tumor removal, with common methods including resection with a blade or direct suction of the tumor.
Cortical stimulation mapping is highly important in this step, where motor function will be assessed frequently throughout the operation from a baseline level prior to the initial incision. This allows for realtime feedback during the surgery to localize the tumor and ensure the surrounding brain tissue’s function is maintained.
During the resection, one or multiple samples of the tissue are collected for pathologic analysis, to identify the specific type of tumor and other studies for further analysis.
Step 7: Closure
After as much of the tumor as possible is safely removed, it is now time to wrap up the procedure. The surgeon will close the dura mater that covers the brain tissue, and then replace the piece of the skull removed, before closing the site surrounding the initial incision.
Step 8: Patient Transport and Post-Operative Care
The patient is then transported from the OR to wherever their preoperative plan indicated, often the neuro ICU or PACU. As the patient wakes from anesthesia and is beginning their recovery, helping them is highly critical to prevent immediate complications surrounding the site of the surgery and allow proper healing.
Sources
[1] https://www.pacehospital.com/brain-tumor-symptoms-causes-complications-prevention-and-treatment
[2] https://www.cancer.gov/rare-brain-spine-tumor/tumors/diffuse-midline-gliomas
[3] https://www.quora.com/How-is-the-head-prevented-from-moving-during-neurosurgery
[6] https://www.frontiersin.org/journals/neurology/articles/10.3389/fneur.2023.1170045/full
[7] https://www.aaroncohen-gadol.com/en/patients/craniotomy/overview
[8] https://www.istockphoto.com/illustrations/patient-transport
[9] https://www.kucancercenter.org/cancer/cancer-types/brain-cancer/brain-cancer-diagnosis-screening