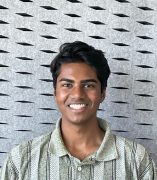
Mehul Patnam
Student Participant
Cardiology
Email:
Week 1: A Balance Between Zeal and Ambition Heading link
My first week of clinical immersion in the Cardiology department was a gratifying, yet daunting experience. In my past when I have spent time in clinical settings, I’ve always felt like a novice in a room full of experts. I’ve always found myself struggling to understand the basic workflow of a hospital setting and identify how to minimize my presence. However, this time it felt natural.
On Tuesday, we started off in the clinic—an ideal way to embark upon our 6-week-long whirlwind tour of cardiology. The slow pace of patient visits allowed ample time for my group members and me to settle into our roles as student observers and connect with our faculty mentor. I felt calm in knowing that I had time to reflect on each patient that I saw before being rushed off to the next one. As much as I struggled to shut off my clinical brain during these patient visits and instead channel my inner engineer, my biggest point of satisfaction from this first week was being able to tie clinical knowledge to the deluge of basic science information from my first year of medical school. My eyes widened in awe with each symptom, drug name, and disease process that I recognized. Seeing my hard work translate into clinical understanding, I could hardly contain the evanescent smirks that kept creeping up on my face. For the first time in a clinical setting, I felt comfortable asking meaningful questions to sate my curiosity, rather than defaulting to silence out of fear.
The rest of the week heralded a gauntlet of technically intensive procedures as part of the electrophysiology (EP) and catheterization (cath) labs. It was during these cases that I sought to engage my engineering brain. What shocked me most was the marked difference between approach to EP and cath lab cases. Though I had envisioned cath lab procedures to be the most time-consuming and arduous, it was actually the opposite. The vast majority of cath lab procedures I observed during this first week were routine, almost as if the clinicians were sticking to a detailed protocol listed in a textbook. Instead, it was the EP procedures which seemed to most engage the clinicians’ brains. The entire procedure seemed to be in flux, with the final decision on what tissue to ablate depending on the results of a 3D voltage mapping of the heart. It was inspiring to watch the physicians in the room discuss surgical strategies for the patient according to their real-time findings throughout the procedure. During these cases, I identified several good and bad design components I encountered in my first week of cardiology:
Good Design: Use of Inari FlowTriever to remove right atrial, pulmonary, or superior vena cava blood clots. I found it hard to believe how efficient and intuitive this device was when it came to the removal of blood clots. First a guide wire was inserted into a preplaced catheter originating from the femoral vein. From here, a tube could be inserted into the clot area to either aspirate out the clot via a vacuum or mechanically remove it via the inflation of metal disks. I was worried about how this method of clot removal would be viable, as it seemed to remove a lot of blood along with the clot. However, upon removal, the clinicals inserted the blood-filled syringe into a filter that had another syringe attached to the other side. This allowed for the separation of the blood clot and the filtered blood to be transfused back into the patient.
Bad Design: Need to continually induce ventricular tachycardia (VT) to identify the origin of aberrant circuits and the specific cardiac tissue to ablate. Though precision voltage mapping of the heart was fascinating and exact, it was an extremely time-consuming procedure in which only one or two of the clinical personnel were actively involved. The attending employed an additional method: inducing VT in a patient suffering from intermittent bouts of VT to identify regions of scar tissue which these aberrant circuits are arising from. Though this method seems to be more time efficient, it carries risks, the most dangerous of which is causing ventricular fibrillation—a potentially deadly arrhythmia. Additionally, constantly inducing and reverting ventricular tachycardia can stun the heart, leading to heart failure. Interestingly, the attending cited similar outcomes in the literature between the precision voltage mapping and inducible ventricular tachycardia methods.
There is so much more I want to see and so much I want to change about what I’ve already encountered. However, I recognize that it’s just the first week, I’ve barely finished my first week of medical school, and there is a long road ahead. I anticipate that I will find difficulty in indulging my own curiosity to identify needs while reigning in my desire to start innovating.
Week 2: Just Burn it All Heading link
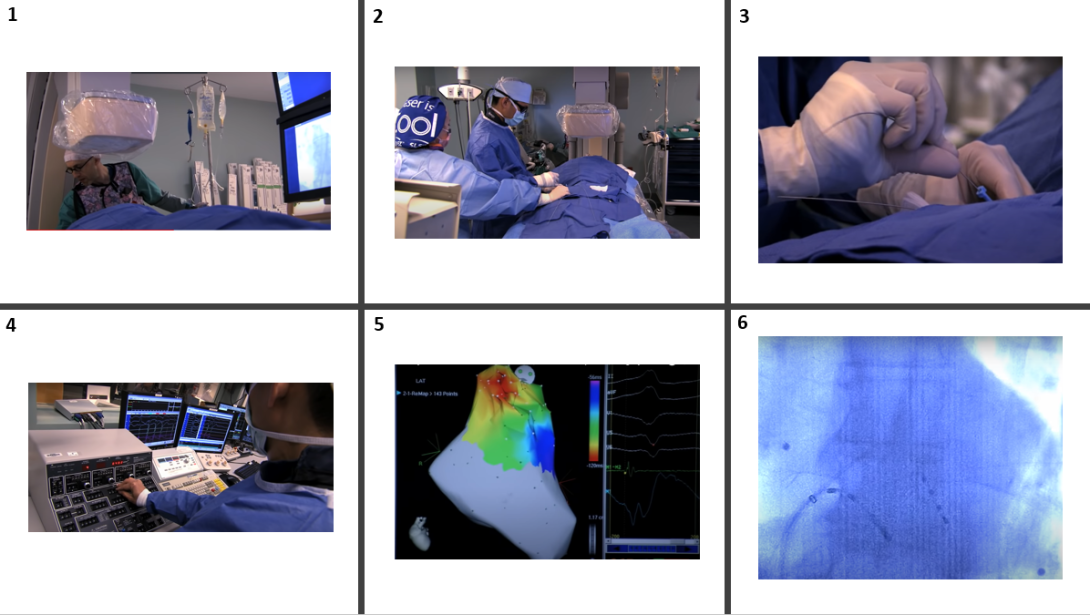
It baffles me how complex arrhythmias can be corrected via a miniscule incision in a patient’s groin. The device that enables such a feat—the catheter—employs ingenious design principles in doing so. The storyboard I chose to map out for this week was one that I touched on in my discussion of bad design last week: ventricular tachycardia ablation. This time, however, I want to highlight the simultaneous intricacy and elegance of such a life-changing procedure.
1: The procedure begins before the electrophysiologist even enters the room, with the anesthesiologist putting the patient to sleep. A potential pain point of this procedure is the decision of what type of anesthesia to use. Both the type of procedure and patient characteristics impact how anesthesia is administered. The goal is to administer the least potent form of anesthesia that will allow for resolution of the procedure without complications. Whereas some intensive procedures may call for intubation and general anesthesia, the anesthesiologists opted for monitored anesthesia care (MAC), in which the patient was sedated, but conscious and aware of their surroundings. To my surprise, the patient would often talk and move throughout the procedure, displacing some of the catheters in the process!
2: The cardiologist makes an incision into the groin and implants a sheath into the femoral vein for catheter access. Potential pain point: bleeding during incision and puncture of surrounding vessels, such as the femoral artery.
3: Insertion of a guide wire and catheters into the sheath. Several catheters can be inserted into the opening created by the sheath, such as those with electrodes at the tips to map voltage and those with the capability of heating up to burn underlying cardiac tissue. Potential pain point: catheters are floppy and can be difficult to maneuver to the desired spot. A solution to this is to reinforce catheters with a stylet inserted into the lumen of the catheter that can be maneuvered from outside the body. However, the catheter/stylet device still carry the risk of perforating cardiac tissue when mapping voltage near the apex or walls.
4: Ventricular tachycardia is induced via electrode stimulation of myocardial cells. Potential pain point: ventricular tachycardia is an abnormal heart rhythm that when constantly induced/reverted can lead to ventricular fibrillation and heart failure.
5: 3D voltage map of the heart is created to identify areas of scar and where the arrhythmia is originating from. Potential pain point: arrhythmia-causing scar tissue may be difficult to locate even with substrate mapping and can be confused with lack of contact between probe and endocardium. This would give off a similar signal to dead tissue (both have no electrical conductance).
6: Ablation area is identified, and catheters are heated up to burn the heart tissue. Potential pain point: despite precision mapping, ablation can cause damage to surrounding tissue. Physicians will also sometimes burn extra tissue to ensure obstruction of the arrhythmia.
Link to Peer-Reviewed Article: https://pubmed.ncbi.nlm.nih.gov/31857035/
The article I chose focused on the history of ventricular tachycardia ablation and touched on emerging novel techniques which might replace current methods in the future. In the past, ventricular tachycardia ablation had to be treated with open heart surgery. However, the advent of catheter-guided procedures removed this restriction. The article gave an extensive overview of four the four mapping mechanisms: activation, entrainment, pace, and substrate. Finally, it touched on new procedures that might replace radiofrequency (RF) ablation, such as alcohol ablation and noninvasive substrate mapping.
Images taken from https://www.youtube.com/watch?v=Y-YzXbfcAas
Week 3: It’s getting hot in here…or just the opposite? Heading link
“I don’t burn, I ablate,” announced the attending physician as he qualified my question about an ablation procedure. I had mistakenly used the word “burn” to refer to his use of a catheter to destroy a piece of myocardium harboring an aberrant electrical circuit. In my defense, in every procedure I had observed since embarking on my whirlwind tour of cardiology, the attending employed heat energy to burn the suspicious heart tissue. I pondered that night about why the attending was so adamant that I do not conflate two terms which I had heard the fellows and medical staff use interchangeably.
“To ablate” means to surgically remove or destroy a section of tissue from the body. Since it is difficult to cut out a piece of heart tissue while remaining minimally invasive, the next logical method to destroy tissue is via burning. Though this is what people first envision when they hear the term “ablate” it is not the only means to destroying heart tissue. It didn’t take long for this realization to dawn upon me. The very first procedure the next morning was an atrial fibrillation ablation that employed a cryoballoon, a device that uses cold energy to damage the faulty myocardium.
The procedure was fascinating, as I was able to visualize the balloon inflating to freeze the tissue surrounding the pulmonary veins via X-ray. I was immediately enthralled by the elegant device, as it completed the ablation in just four freezes, compared to the cumbersome radiofrequency (RF) catheter that took many more burns and extreme precision to operate. For context, the RF catheter can move if the patient moves or if the physician’s hand slips. Instead, the cryoballoon acts like a popsicle once frozen, sticking to the warmer tissue of the endocardium and allowing for stable destruction of the tissue surrounding the pulmonary veins.
Following the procedure, the attending removed the cryoballoon and initiated a freeze outside of the body to show us what was actually happening. As the balloon expanded and became coated in thick layers of ice, I found myself struggling to believe how such a device could operate inside the body, despite the fact that I had just witnessed the procedure! Immediately, my mind began thinking of potential complications. There was a moment in the procedure where the attending paused and seemed to become tense. In this time, he devoted all of his attention to the patient, the screen in front of him, and his surgical staff. Later, I realized that the attending was worried about damaging the phrenic nerve, a structure that innervates the diaphragm and lies near the areas of destruction. Damaging the phrenic nerve can cause shortness of breath in patients for up to a year post operatively, with a small proportion of patients sustaining permanent damage.
The attending employed a couple methods to assess phrenic nerve function and prevent damage. For example, one method the attending mentioned was to pace the phrenic nerve to induce hiccups (via stimulation of the diaphragm). If the hiccups became fainter or stopped, then there might be damage to the phrenic nerve. The problem with this solution is that it doesn’t allow for prevention of damage. Another method the attending employed was to place EKG electrode over the diaphragm to indirectly measure phrenic function. The problem with his however, is that phrenic nerve injury can look similar to the displacement of the electrode-containing catheter—both present with a loss of signal. After observing these methods and seeing the tension in the room during this part of the procedure, I curated the following needs statements:
- Electrophysiologists need a better way to minimize damage to peripheral structures during ablation procedures.
I definitely bit off a lot more than I can chew with this first needs statement. I felt as though I had identified a good population and general problem space, but much refinement was still needed.
- Electrophysiologists who perform cryoballoon ablation for atrial fibrillation need a better way to assess status of the phrenic nerve to prevent damage.
In the second iteration, I specified the procedure in which the complication occurred. There were already methods of risk assessment in place, but the attending mentioned that these are not always perfect, so I decided to focus on risk mitigation.
- Electrophysiologists who perform cryoballoon ablation for atrial fibrillation need to avoid damage to the phrenic nerve in order to reduce incidence of post-operative dyspnea.
In the third iteration of my needs statements, I attempted to continue focusing on the specific population of electrophysiologists, maintain an appropriate scope of my problem space, and state a quantifiable outcome. I realized that more important than assessing status is the ultimate goal of reducing complications, and didn’t want to pigeonhole myself into creating an entirely new diagnostic method for phrenic nerve function. One way of avoiding damage is by better assessing status such that physicians don’t have to stress as much during this portion of the procedure. “Avoid damage to the phrenic nerve” might still be too vague, and thus might benefit from the previous needs statement’s way of focusing on risk assessment.
- Electrophysiologists who perform cryoballoon ablation for atrial fibrillation need a better way to assess status of the phrenic nerve to substitute for currently employed time-consuming and stress-inducing checks.
This final needs statement takes an alternative route. Rather than focusing on the clinical complications, it instead addresses workflow and efficiency in the cath lab. Specifically, there is a problem of cumbersome status checks that are currently used to assess phrenic nerve status. Thus, it aims to equip the physician with an improved tool for measuring phrenic nerve status, rather than building a device to prevent nerve damage in the first place.
Week 4: Solving one problem by creating another Heading link
This week, we spent the majority of our time observing cardiac catheterization procedures in the cath lab—a fresh swap from our typical focus on electrophysiology. We saw several procedures, including several angiographies, a peripheral angiography, and even a stent placement. Throughout all of this variety, however, one aspect remained constant—the employment of a transradial catheter approach. Regardless of whether the procedure being performed was diagnostic or interventional, the physicians and fellows always opted for the transradial approach due to its lower complication rate.
Near the end of the procedure, I noticed the fellows take extra care with one of the last steps they completed. In order to close the radial artery access, the doctors used a syringe to push air into the catheter opening site, introducing a backwards pressure gradient into the radial artery and preventing outward blood flow. While I had thought of this solution as an elegant and ingenious one, the fellows were quick to point out its faults. An extreme level of precision is needed to introduce only the minimal amount of air into the radial artery. The introduction of air and blockage of the radial artery like this increases the risk for radial artery occlusion. When more air is introduced than necessary, this risk increases. Identifying a potential problem space, I came up with the following needs statement:
Cardiologists who perform transradial catheterization procedures need a better way to close off the radial artery following a procedure while minimizing the incidence of future radial artery occlusion.
Keeping the current solution of air-introduction and establishment of a pressure gradient in mind, I delved into the patent literature and found a novel device addressing radial artery closure.
Patent #: US20160213373A1
Title: Radial Artery Closure Device
Description:
This patent describes a device that employs a compression force against the radial artery for closure post-operatively. Unique to this device is an additional compression element that imposes a force against the ulnar artery. A reduction in arterial blood flow to the hand via the ulnar artery causes a compensatory increase in flow to the other major artery that supplies the hand—the radial artery. Thus, by simultaneously compressing the ulnar artery, the device increases and maintains flow in the compressed radial artery, preventing likelihood of radial artery occlusion while maintaining hemostasis.
Claims:
The major claim of this patent is a radial artery closure device that consists of a support plate for housing, a holding strap, a compression surface, a compression element that provides the compressive force to the access site, and a second set of compression elements to compress the ulnar artery. The patent claims to work by first placing the support plate at the access site, partially occluding the ulnar artery, and only then compressing the radial artery. Finally, the device rests on the principle that these two arteries have collateral blood vessels connecting their arterial beds.
Analysis:
This solution is a simple and elegant one. Rather than employing an extrinsic factor of a backwards pressure gradient, it exploits the physiological principles of hyperemia and collateral circulation to create a more natural solution. However, this solution may introduce another problem. In increasing radial blood flow, the device requires compression of the ulnar artery, which could potentially cause the same phenomenon of arterial occlusion, just in a different vessel.
Week 5: Pace Yourself! Heading link
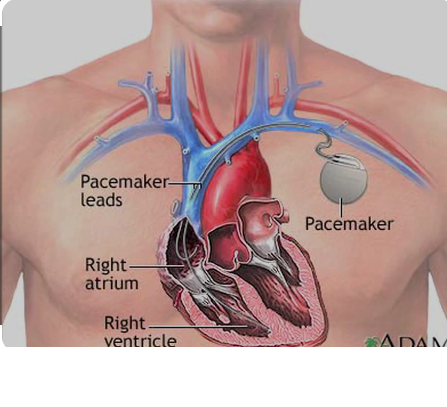
This week, we were tasked with conceptualizing a Total Addressable Market (TAM) for a unique device and needs statement. I decided to pursue the area of pacemaker implantation and replacement. Pacemakers are electrical devices inserted into patients subcutaneously. They aid in regulating abnormal heart rhythms, such as in patients with sinus node dysfunction or specific types of AV heart blocks. A pacemaker can detect when heart rate drops below a certain threshold value and deliver a shock to the patient’s heart, stimulating it and reverting the patient’s heartbeat to normal sinus rhythm. Currently, pacemakers have a battery life of about 5-10 years. Nearing the end of the device’s lifetime, a pacemaker replacement surgery must be performed. Despite being a relatively routine procedure, complications can arise. In the pacemaker replacement, the generator is removed and replaced, while the leads are kept in place. Just as any invasive procedure involving foreign body implantation, the risk of infection is high. Longer battery lives of pacemakers could mean fewer pacemaker replacement surgeries required and ultimately a lower complication/infection rate.
Many patients report not needed their pacemakers during the first 5-10 years, leading to reluctance when the time for battery replacement comes along. Despite this initially quiescent period of the first pacemaker implantation, many of these patients have a pacemaker activation during the second lifetime, underscoring how crucial pacemaker replacement is. Extending battery life, therefore, would not only decrease cost of replacement procedures and infection rates, but also ensure that more patients who need a pacemaker always have one inserted.
In calculating the TAM for pacemaker replacement, I found that approximately 200,000 pacemakers are implanted in the United States annually. In each of these procedures there exists a risk for infection and other complications. The cost of pacemaker replacement is about $15,000. Thus, the TAM for pacemaker replacement = 200,000 * $15,000 = $3 billion.
Week 6: Final Insights Heading link
Six weeks shadowing in the Clinical Immersion Program fueled my interest in cardiology and drive for innovation in a way I could have never imagined. Using my clinical background helped me understand the details of catheter-based procedures, while engaging my engineering brain helped me identify and validate needs. Being in such a foreign environment for the first time was tough, but balancing between my clinical and engineering backgrounds soon became trivial. The first few weeks in particular were the most valuable to me—I felt that behind each corner I turned was a new valuable nugget of information for me to absorb.
My advice to new students in CIP is simple: take it slow and remember to have fun. I recollect that during the first week I was scrambling around the cath lab and even clinic desperate to identify needs, even though I had already identified several other problem spaces. Don’t force yourself to identify needs in situations where a true problem space doesn’t exist. I found that the best problem spaces that we identified arose naturally. At the same time, don’t brush off what you identify as problematic because a clinician assures you it is not a critical issue. Sometimes, what may seem like a small inconvenience for a physician may be the tip of the iceberg masking more pressing, universal problems.